Fire ants build towers with three simple rules
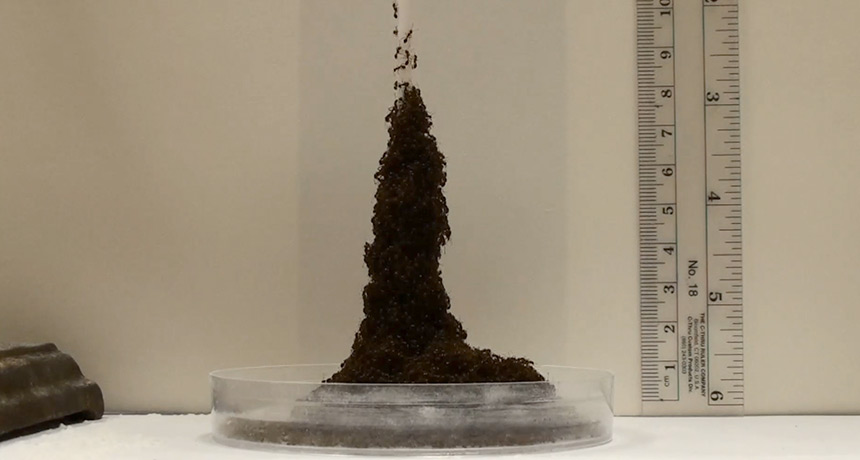
When faced with rushing floodwaters, fire ants are known to build two types of structures. A quickly formed raft lets the insects float to safety. And once they find a branch or tree to hold on to, the ants might form a tower up to 30 ants high, with eggs, brood and queen tucked safely inside. Neither structure requires a set of plans or a foreman ant leading the construction, though. Instead, both structures form by three simple rules:
If you have an ant or ants on top of you, don’t move.
If you’re standing on top of ants, keep moving a short distance in any direction.
If you find a space next to ants that aren’t moving, occupy that space and link up.
“When in water, these rules dictate [fire ants] to build rafts, and the same rules dictate them to build towers when they are around a stem [or] branch,” notes Sulisay Phonekeo of the Georgia Institute of Technology in Atlanta. He led the new study, published July 12 in Royal Society Open Science.
To study the fire ants’ construction capabilities, Phonekeo and his Georgia Tech colleagues collected ants from roadsides near Atlanta. While covered in protective gear, the researchers dug up ant mounds and placed them in buckets lined with talc powder so the insects couldn’t climb out. Being quick was a necessity because “once you start digging, they’ll … go on attack mode,” Phonekeo says. The researchers then slowly flooded the bucket until the ants floated out of the dirt and formed a raft that could be easily scooped out.
In the lab, the researchers placed ants in a dish with a central support, then filmed the insects as they formed a tower. The support had to be covered with Teflon, which the ants could grab onto but not climb without help. Over about 25 minutes, the ants would form a tower stretching up to 30 mm high. (The ants themselves are only 2 to 6 mm long.)
The towers looked like the Eiffel Tower or the end of a trombone, with a wide base and narrow top. And the towers weren’t static, like rafts of ants are. Instead, videos of the ant towers showed that the towers were constantly sinking and being rebuilt.
Peering into the transparent Petri dish from below revealed that the ants build tunnels in the base of a tower, which they use to exit the base before climbing back up the outside.
“The ants clear a path through the ants underneath much like clearing soil,” Phonekeo says. Ants may be using the tunnels to remove debris from inside the towers. And the constant sinking and rebuilding may give the ants a chance to rest without the weight of any compatriots on their backs, he says.
To find out what was happening inside the tower, the researchers fed half their ants a liquid laced with radioactive iodide and then filmed the insects using a camera that captured X-rays. In the film, radioactive ants appeared as dark dots, and the researchers could see that some of those dots didn’t move, but others did.
The team then turned to the three rules that fire ants follow when building a raft and realized that they also applied to towers. But there was also a fourth rule: A tower’s stability depends on the ants that have attached themselves to the rod. The top row of ants on the rod aren’t stable unless they form a complete ring. So to get a taller tower, there needs to be a full ring of ants gripping to the rod and each other.
That such simple rules could form two completely different structures is inspiring to Phonekeo. “It makes me wonder about the possibilities of living structures that these ants can build if we can design the right environment for them.”