Two artificial sweeteners together take the bitter out of bittersweet
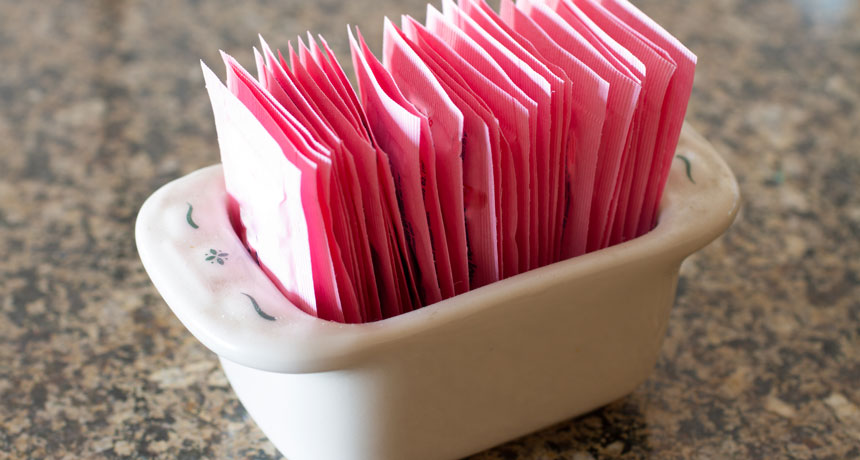
Artificial sweeteners can have a not-so-sweet side — a bitter aftertaste. The flavor can be such a turnoff that some people avoid the additives entirely. Decades ago, people noticed that for two artificial sweeteners — saccharin and cyclamate, which can taste bitter on their own — the bitterness disappears when they’re combined. But no one really knew why.
It turns out that saccharin doesn’t just activate sweet taste receptors, it also blocks bitter ones — the same bitter taste receptors that cyclamate activates. And the reverse is true, too. The result could make your bitter batter better. And it could help scientists find the next super sweetener.
Saccharin is 300 times as sweet as sugar, and cyclamate is 30 to 40 times as sweet as the real deal. Saccharin has been in use since its discovery in 1879 and is best known as Sweet’N Low in the United States. Cyclamate was initially approved in the United States in 1951, but banned as a food additive in 1969 over concerns that it caused cancer in rats. It remains popular elsewhere, and is the sweetener behind Sweet’N Low in Canada.
In the 1950s, scientists realized that the combination of the two (sold in Europe under brand names such as Assugrin), wasn’t nearly as bitter as either sweetener alone.
But for the more than 60 years since, scientists didn’t know why the combination of cyclamate and saccharin was such a sweet deal. That’s in large part because scientists simply didn’t know a lot about how we taste. The receptors for bitter flavors were only discovered in 2000, explains Maik Behrens, a molecular biologist at the German Institute of Human Nutrition Potsdam-Rehbruecke.
(Now we know that that there are 25 potential bitter taste receptors, and that people express them in varying levels. That’s why some people have strong responses to bitter flavors such as those in coffee, while others might be more bothered by the bitter aftertaste of the sweetener put in it.)
Behrens and his colleagues Kristina Blank and Wolfgang Meyerhof developed a way to screen which of the bitter taste receptors that saccharin and cyclamate were hitting, to figure out why the combination is more palatable than either one alone. The researchers inserted the genes for the 25 subtypes into human kidney cells (an easier feat than working with real taste cells). Each gene included a marker that glowed when the receptors were stimulated.
Previous studies of the two sweeteners had shown that saccharin alone activates the subtypes TAS2R31 and TAS2R43, and cyclamate tickles TAS2R1 and TAS2R38. Stimulating any of those four taste receptor subtypes will leave a bitter taste in your mouth.
But cyclamate doesn’t just activate the two bitter receptors, Behrens and his colleagues showed. It blocks TAS2R31 and TAS2R43 — the same receptors that saccharin stimulates. So with cyclamate around, saccharin can’t get at the bitter taste subtypes, Behrens explains. Bye, bye bitter aftertaste.
The reverse was true, too: Saccharin blocked TAS2R1 — one of the bitter receptors that cyclamate activates. In this case, though, the amount of saccharin required to block the receptors that cyclamate activates would have bitter effects on its own. So it’s probably the actions of cyclamate at saccharin’s bitter receptors that help block the bitterness, Behrens and his colleagues report September 14 in Cell Chemical Biology.
The researchers also tested whether cyclamate and saccharin together could be stronger activators of sweet receptors than either chemical alone. But in further tests, Behrens and his colleagues showed that, no, the sweet sides of saccharin and cyclamate stayed the same in combination.
“This addresses a longstanding puzzle why mixing two different sweeteners changes the aftertaste,” says Yuki Oka, a neuroscientist at Caltech in Pasadena. “They are interrupting each other at the receptor level.” It’s not too surprising that a sweetener might block some receptor subtypes and stimulate others, he notes, but that saccharin and cyclamate have such clear compatibilities is a lucky chance. “Mechanism-wise, it’s surprisingly beautiful.”
Oka notes that no actual tongues tasted artificial sweeteners in these experiments. The tests took place on cells in dishes. But, he says, because the researchers used the human bitter taste receptors, it’s likely that the same thing happens when a diet drink hits the human tongue.
Behrens hopes the cell setup they used for this experiment can do more than solve an old mystery. By using cells in lab tests to predict how different additives might interact, he notes, scientists can develop sweeteners with fewer bitter effects. The technique was developed with funding from a multinational group of researchers and companies — many of which will probably be very interested in the sweet results. And on the way to sweeteners of the future, scientists may be able to resolve more taste mysteries of the past.